Optimal detection of spatial modulated signals in 5G and beyond: Agorithms and hardware architectures
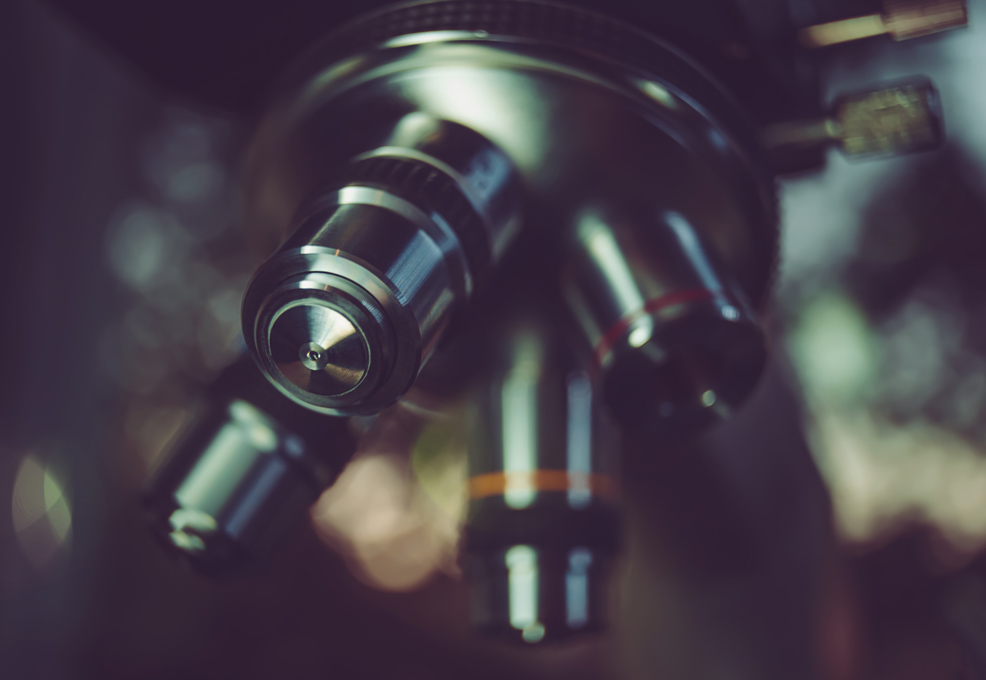
Author(s)
Che-Hsin LinBiography
Che-Hsin Lin is currently a distinguished research professor of National Sun Yat-sen University, Taiwan and a chair professor of the ASE Groups. He has served as the technical program committee (TPC) member for MicroTAS, IEEE MEMS and Transducers, and the IEEE NEMS conferences. His research interests are in MEMS fabrication technologies, bio-MEMS, microfluidic systems, bio-photonics and the applications of atmospheric plasma.
Academy/University/Organization
National Sun Yat-sen University-
TAGS
-
Share this article
You are free to share this article under the Attribution 4.0 International license
- ENGINEERING & TECHNOLOGIES
- Text & Image
- January 21,2020
The optical microscope was designed and used by Robert Hooke in 1665. Optical microscopes play the most important role in the observations and recordings of small objects in the biological and life sciences. Over the next 300 years, scientists greatly improved optical microscopes and invented various microscopes by using higher quality lenses and better illumination methods. However, these conventional microscopes were designed according to a finite-tube length scheme, i.e., an intermediate image is formed between the objective lens and eyepiece. This finite-tube scheme is straightforward and simple but is not flexible since the distance between the objective and the eyepiece is fixed. During the past decades, a new infinite-tube length scheme was developed and produced by the major optical microscope manufacturers. This infinity-tube scheme saves space for scientists to add various kinds of optical components for different applications since light propagates parallel in the tube due to the insertion of a tube lens between the objective and the eyepiece. Prof. Che-Hsin Lin of BEMS Lab. in the National Sun Yat-sen University has developed a number of advanced optical microscope systems for various bio-chip detection applications including a TIRF microscope system for Joule heat measurement, a confocal optical tweezers system for cell counting and sorting, a diascopic illumination scheme for simultaneous multi-spectral fluorescence detection, a chromatic aberration for rapid depth detection of moving objects, and a TIRF objective SPR system for high spatial resolution detection.
In 2015, people celebrated the 350th anniversary of the publication of Micrographia by Robert Hooke. For people living in the mid-seventeenth century, Micrographia helped them to see large-scale illustrations of tiny creatures from everyday life such as fleas, mites, ants and other bugs. Obviously, the optical microscope has greatly extended humans’ vision since the compound microscope was invented in 1665. With the emerging needs and rapid development of lens grinding techniques, a great number of microscopes have been developed including the stereo microscope, the fluorescence microscope, the phase contrast microscope, the dark field microscope, the total internal reflection microscope and the confocal microscope. However, the rapid development of these modern microscope systems relied on the infinity corrected optical design. The infinity-corrected optical system is not used in commercial microscopes but only for research and industrial optical systems. Figure 1 shows a comparison of the light paths of the conventional finite-tube microscope and the modern infinity-tube microscope. An infinity-corrected optical system has the following advantages because a parallel light beam passes between the objective lens and tube lens. A magnification does not change even if the distance between the objective lens and tube lens is changed. Therefore, various optical components and adapters can be inserted between the objective and tube lens without interfering with the image formation at the eyepiece. Scientists have this space to construct microscopes of different functions.
Figure 1. Light paths in the conventional finite-tube microscope and the modern infinity-tube microscope.
Microfluidic bio-chips are miniaturized fluidic devices which are capable of rapid and accurate analysis of small amounts of biological samples, such as DNA, protein, cells, pathogens, etc. in a short period of time. The microfluidic bio-chip is one of the most promising techniques for developing the IVD (in-vitro diagnostic devices) for clinical detection. Prof. Che-Hsin Lin, a distinguished professor in NSYSU, Kaohsiung, has more than 18 years of experience in microfluidic bio-chips. He is also a lover of photography optics and owns a number of camera bodies and lenses. The mixing of his personal interest and hands-on experience with optical microscopes has induced numerous new ideas for “playing with the microscope optics.” Microscopes play an important role in the observation and analysis of the microfluidic bio-chips due to the small feature size of the microfluidic channels. The modern infinity-tube microscope design has brought numerous possibilities for integrating modern optics and microfluidic devices. With the mixing of interest in photography, a passion for doing research and some crazy ideas, Prof. Lin has developed several innovative microscope schemes for high performance microfluidic bio-chip detection, and has published several research papers in the field of bio-photonics. Every purchased microscope in his laboratory was disassembled for structure modification to add new functions immediate upon its arrival.
Figure 2. Bio-photonics mixes the microfluidic chip, advanced microscope schemes and personal interests, passions and crazy ideas.
For example, he developed a new white-light TIRF (total-internal reflection fluorescence) system combining the temperature-sensitive fluorescence dye, Rhodamine B, to measure the temperature distribution in a 100-m wide microchannel. This work was reported in the journal of Microfluidics and Nanofluidics [1]. The microscope system was used for image observation and temperature distribution measurement at the solid/liquid interface. In addition, the single wavelength laser or the color-filtered Hg lamp are the light source in the typical fluorescence microscope. This fluorescence detection scheme is usually for single color detection and limits the throughput of a fluorescence microscope for bio-chip detection. By adapting a light splitting port for the infinity-tube microscope, a low-cost commercial liquid crystal device (LCD) projector with digitally-modulated LCD switches is used to provide the illumination light source, and the fluorescence emitted from the CE chip is synchronously detected using an ultraviolet–visible–near-infrared (UV–vis–NIR) spectrometer. With this approach, a multi-wavelength capillary-electrophoresis detection was achieved within a single channel, and a single test run was performed without needing to move the optical components [2]. Parallel multi-wavelength detections always attract researchers to these bio-chips. In this regard, Prof. Lin also developed a novel “diascopic illumination scheme” for multi-wavelength fluorescence detection [3, 4]. Alternatively, it is quite challenging to detect the depth position of rapidly moving cells or particles inside an embedded microfluidic channel. With a similar diascopic illumination scheme, a plastic lens was inserted into the light path to produce “chromatic aberration” in the microscope for detecting the depth position of rapidly moving objects flowing in a microfluidic channel [5]. As shown in Fig. 3, this is an absolutely crazy idea in the design of an optic system since chromatic aberration is generally an unwelcome effect in optics and should be eliminated by applying the expensive low-aberration lenses in the light path. However, Prof. Lin successfully turned the drawback effect into a positive principle, and demonstrated high performance sensing results. These examples including the ideal of combining the microfluidic bio-chips and modern microscopes do not limit the passion and imagination of Prof. Lin for constructing interesting optical detection schemes in bio-photonics.
Figure 3 (A) Basic concept of particle depth detection by means of the chromatic aberration effect. (B) Schematic illustration of the experimental measurement system. Note that a low Abbe number acrylic glass lens is used to disperse the white light (Lens 1), a high N.A. objective lens is used to condense the dispersed light, and a low N.A. objective lens is used to collect the scattered light. [5]
Reference:
1.DOI: 10.1007/s10404-008-0328-y
2.DOI: 10.1016/j.bios.2010.06.056
3.DOI: 10.1109/JSEN.2011.2170061
4.DOI: 10.1016/j.bios.2009.07.036
5.DOI: 10.1109/JLT.2013.2243818
STAY CONNECTED. SUBSCRIBE TO OUR NEWSLETTER.
Add your information below to receive daily updates.