In Search of Treatment for COVID-19 – Synthesis of Remdesivir and Development of Antiviral Drugs in Academia Sinica
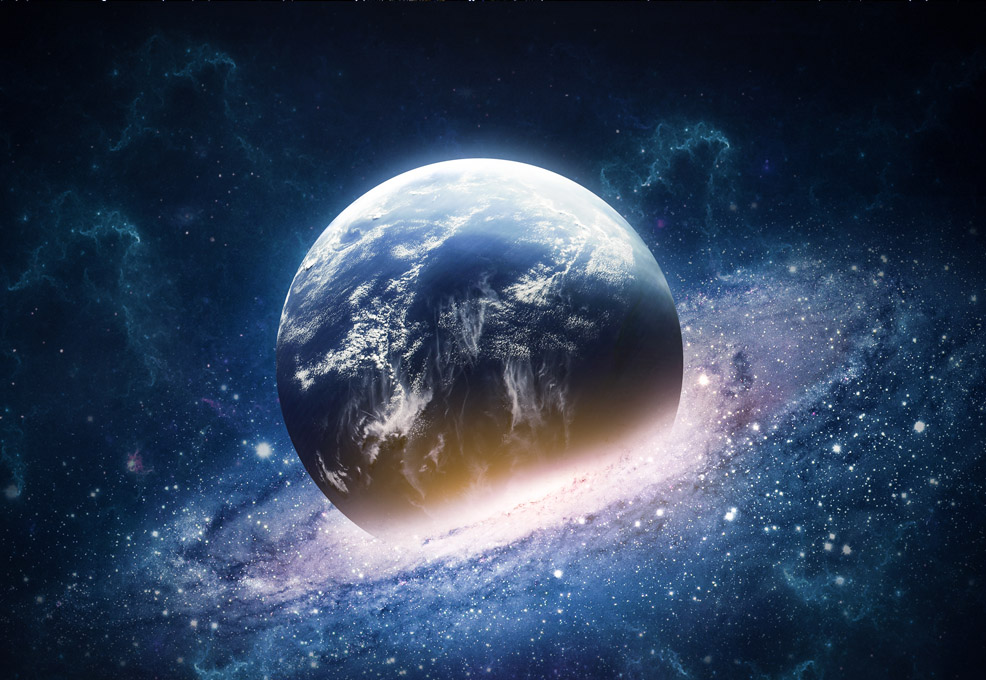
Author(s)
Min-Kai LinBiography
Dr. Lin is currently an assistant research fellow at the Academia Sinica Institute of Astronomy and Astrophysics (ASIAA). He previously worked as a postdoctoral fellow at the Canadian Institute for Theoretical Astrophysics and as the Steward Theory fellow at the University of Arizona. He obtained both his undergraduate and graduate degrees in astronomy from the University of Cambridge.
Academy/University/Organization
Academia Sinica-
TAGS
-
Share this article
You are free to share this article under the Attribution 4.0 International license
- NATURAL SCIENCES
- Text & Image
- May 22,2019
We are not alone. To date, thousands of planets have been discovered beyond our own Solar System. Planets are believed to form in disks of rotating gas and dust around young stars called protoplanetary disks (PPDs). In the mainstream, “bottom-up” scenario, small dust particles aggregate to form intermediate sized planetesimals, before being assembled into planets. However, this picture is complicated by the interaction with gas in PPDs. Thus, we have developed a simple model to describe the complex dust-gas interaction in PPDs, and have applied it to study key problems in the early epochs of planet formation.
Where do we come from? This is a question that everyone asks at some point in their lives. However, this apparently innocent question has puzzled humankind since the dawn of time. It is a mystery that transcends age, gender, race, culture, and history. It is a simple question, yet the answer is far from so.
In order to trace the origin of life, we need to first understand how our home — Earth — was formed. This, in turn, requires us to study the formation of the Solar System. In fact, as of May 2019, there are over 4,000 planets in over 3,000 planetary systems outside the Solar System. Planets appear to be ubiquitous in the Universe. While the details of planet formation remain elusive, we have a rough idea of the overall picture. Planets are believed to form in disks of gas and dust surrounding young stars. Recent observations of these protoplanetary disks (PPDs) indeed reveal detailed structures that hint at ongoing planet formation.
In the mainstream ‘core accretion’ scenario, planets are formed in PPDs via the coagulation of km or larger sized planetesimals through gravity. However, solids in PPDs begin as micron-sized dust particles. How do they grow into the building blocks of planets? These dust particles are immersed in a gaseous environment. Thus, to study planetesimal formation we need to model the interaction between gas and dust. Current state-of-the-art computer simulations follow the evolution of individual dust particles as they traverse the gaseous PPD. This approach, while realistic, is often expensive and the results are sometimes difficult to interpret in a straightforward way. It would therefore be useful to have a simpler model of dusty-gas in PPDs.
To this end, we have developed a model of dusty gas that captures the essential features of dust-gas dynamics in PPDs without the complexity or expense of explicitly following the dust species (Lin & Youdin, 2017; Fig. 1). Our model is best applied to the small particles in the outer parts of PPDs. With this limit, we showed that the two-phase, dusty-gas disk is equivalent to a single-fluid with a special cooling law. The model closely resembles those in textbooks in fluid dynamics. Its simplicity allows it to be readily implemented in computer codes to simulate dusty PPDs. Furthermore, its connection to standard fluid dynamics allows us to understand dusty gas phenomena in terms of hydrodynamics, and to discover dusty analogs of hydrodynamic phenomena. We have applied this new framework to study two basic problems in planet formation theory, as described below.
.jpg)
Figure 1. We have developed a hydrodynamic framework to study the dynamics of dusty gas. We demonstrated a physical analogy between dusty gas (left) and pure gas dynamics (right). This connection allows one to study dusty-gas in PPDs using the standard equations of fluid dynamics. Lin & Youdin (2017)
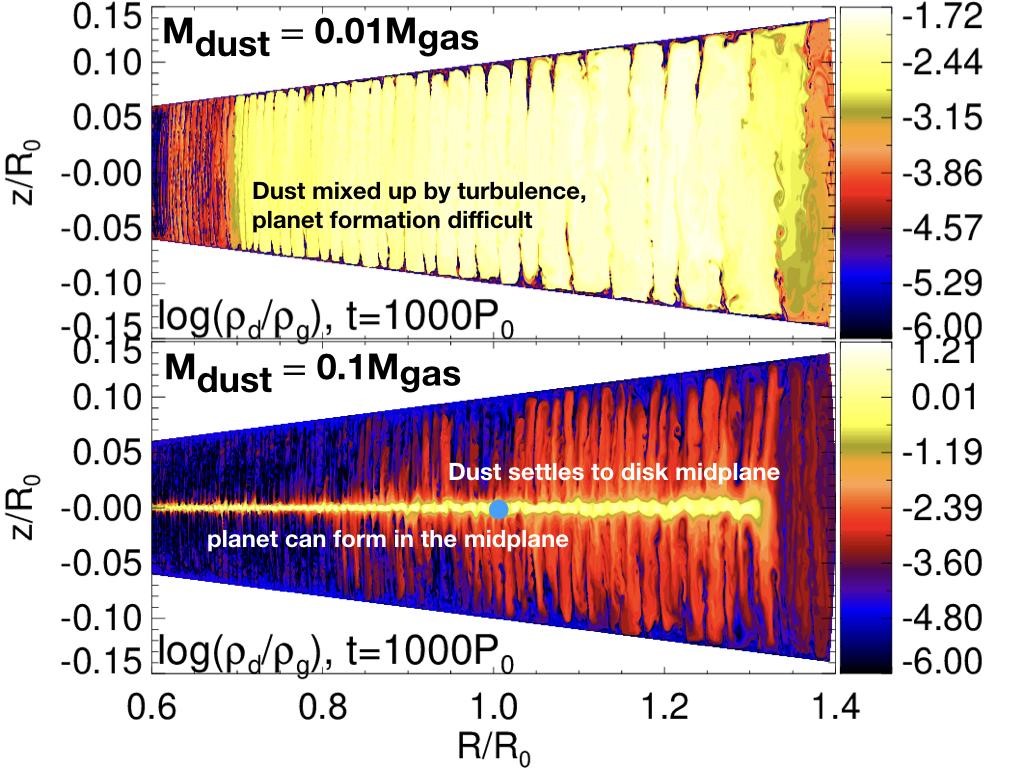
Figure 2. Numerical simulation of dust settling in turbulent PPDs. In the top panel the solid-to-gas mass ratio is 1%, a value typical of the ISM. In the bottom panel, this ratio is 10%. At low solid abundance turbulence stirs up dust particles. Increasing the solid abundance allows particles to form a dense midplane, which is favorable for planet formation. Adapted from Lin (2019).
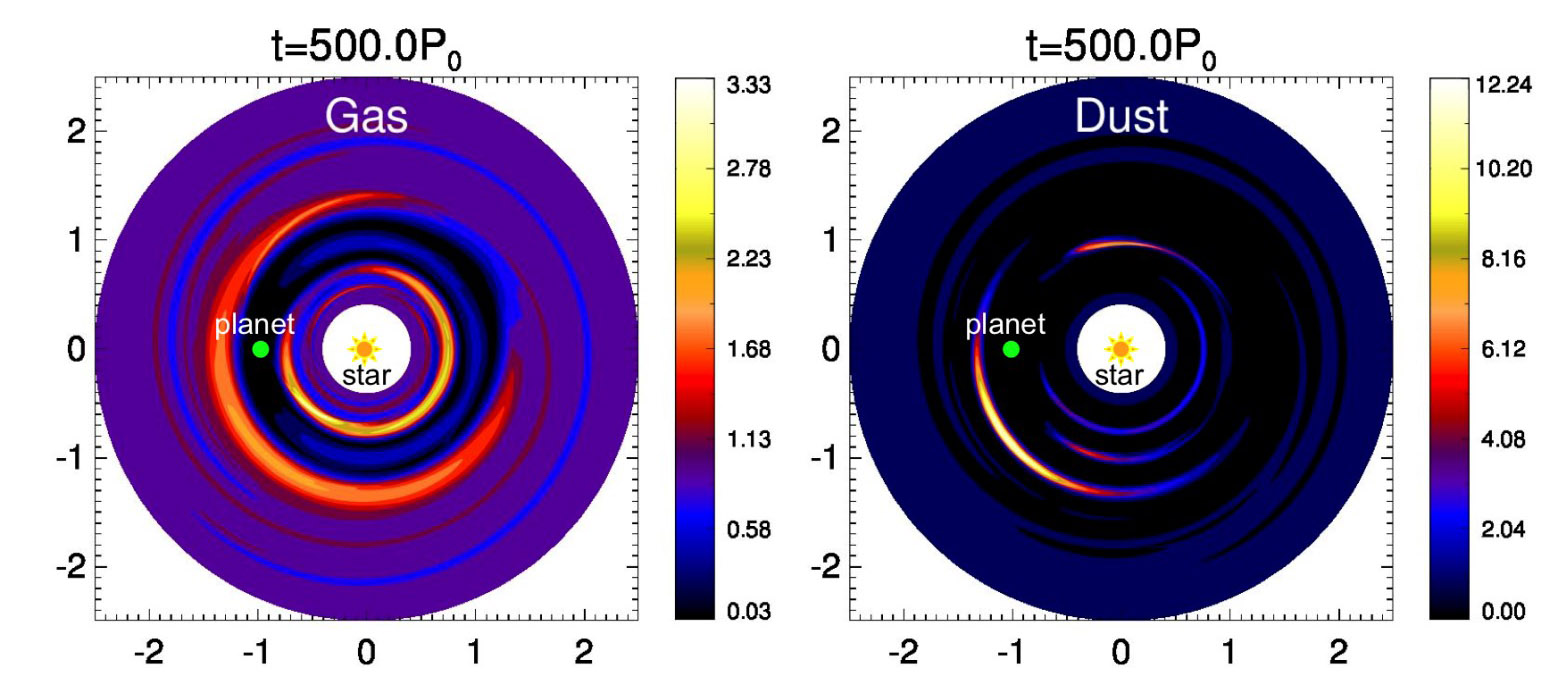
Figure 3. Numerical simulation of disk-planet interaction. A massive planet in a PPD opens up a density gap in the disk, which subsequently becomes unstable and develops a large-scale vortex that act as dust traps (at 7 o’clock). The colorbar shows the surface density of gas (left) and dust (right) normalized by their initial values. Adapted from Chen & Lin (2018).
STAY CONNECTED. SUBSCRIBE TO OUR NEWSLETTER.
Add your information below to receive daily updates.