DNA Biopolymer Photonics:An Innovative Platform Toward Sustainable Technology
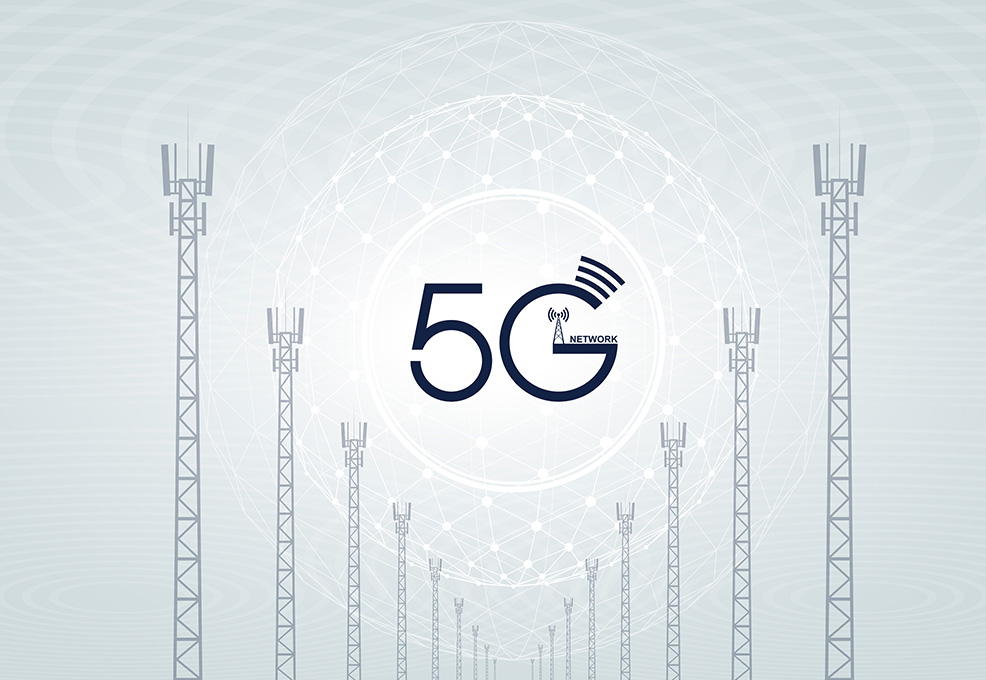
Author(s)
Tsung-Hsien LiuBiography
Prof. Liu is with the Department of Communications/Electrical Engineering, National Chung Cheng University, Chia-Yi, Taiwan. His research interests lie in the area of applying signal processing techniques to wireless communications, with emphasis on developing fast implementation algorithms, designing baseband hardware architectures, and analyzing statistical performance for communication receivers.
Academy/University/Organization
National Chung Cheng University-
TAGS
-
Share this article
You are free to share this article under the Attribution 4.0 International license
- ENGINEERING & TECHNOLOGIES
- Text & Image
- March 17,2021
The 5G communications and beyond will allow us to transmit information with much higher data rate. Several communication baseband technologies, including the spatial modulation (SM), have been proposed to achieve this goal. This promising spatial modulation is applicable when multiple antennas are deployed at both the transmitter and receiver sides. The expensive radio frequency chains required by this technology is few, the feature of which leads the communication devices that adopt this technology to be low-cost. One major challenge for this SM scheme is to design and realize low-cost receiver with good performance. We have designed one optimal hardware detector, in the maximum likelihood detection sense, for the spatial modulation signals. We also have designed one enhanced and nearly optimal hardware detector for the generalized spatial modulation signals. Our results in the past two years show that low-cost and hardware-efficient detector for the nearly optimal generalized spatial modulation is possible. Our detector is able to provide data throughput rate over 1 Gbps under the TSMC 90 nm CMOS technology, while operating at frequency 322.6 MHz. These important and pioneering results may support the spatial modulation as a strong competitor for the future 5G and beyond.
Chip layout and gate counts of each functional block for the proposed GenSM detector.
The 5G communications and beyond will allow us to transmit information with much higher data rate. Several communication baseband technologies, including the spatial modulation (SM), have been proposed to achieve this goal. Recently, the use of high frequency carriers allows the communication transmitter to have a small number of expensive radio-frequency (RF) chains and a large number of antennas deployed in a small area. The SM scheme (see Fig. 1) is target at such transmitter scenarios. For the SM scheme, each block of information bits is mapped to a selected antenna and a symbol (see Fig. 2). The SM symbol is passed to the single RF chain and the selected antenna for transmission. The transmitter adopting the SM scheme is therefore able to transmit data with higher data rate, because it transmits additionally the data bits associated with index of the selected antenna.
Figure 1. The MIMO system with SM scheme.
Figure 2. One example of the SM scheme to map bits to SM symbols.
To achieve the optimal maximum likelihood detector (MLD), we derived a new mathematical formula as a new MLD solution. To avoid the numerically unstable division operation in the hardware detector, we applied Givens rotation to realize the proposed MLD and obtained a low-complexity MLD algorithm. We then followed to propose a coordinate rotation digital computer (CORDIC) based hardware architecture to detect SM signals with 64-quaternary amplitude modulated (QAM) symbols over the multiple input multiple output (MIMO) correlated channel with 8 transmit antennas and 4 receive antennas. Under the TSMC 90-nm CMOS technology, the VLSI implementation results for our designed SM hardware detector reveal that while operating at frequency 384.6 MHz, our CORDIC based architecture requires 70.5K equivalent gates and processes data at detection throughput 432.68 Mbps. Our hardware architecture is the first one to achieve exactly the MLD performance. Our detector can be easily extended to detect SM signals with arbitrary M-ary QAM symbols and arbitrary numbers of transmit and receive antennas.
Figure 3. The proposed hardware architecture for the GenSM detector.
Furthermore, the antenna selection scheme is also combined with the spatial multiplexing (SMX) MIMO scheme to become the general spatial modulation (GenSM). The GenSM scheme is to map each block of data bits to a set of SMX symbols and an index of transmit antenna combination (TAC) of selected antennas. The SMX symbols are then transmitted in parallel through the multiple selected antennas. The number of transmit RF chains is equal to the number of SMX symbols. Due the multiple SMX symbols and higher TACs, the GenSM is able to provide higher data rate than the SM. In the meanwhile, the detection of the GSM signals is more challenging than the detection of SM signals, and, needs to recover the SMX symbols as well as the TAC index. To solve the problem of optimal MLD of GenSM signals with low complexity, two previous algorithms revised the computationally efficient sphere decoding algorithm (SDA) for detecting SMX signals in the original SMX MIMO system. The two algorithms needed, during tree traversals, to discriminate the SMX symbols from the null symbols transmitted by inactive antennas. The computational complexity of this work is formidably high. In our work, we proposed a new sorting assisted successive SDA (SA-SSDA) that employed SDA for a couple of times though to avoid the discrimination of the null symbols from SMX symbols during tree traversals. Particularly, our SA-SSDA takes advantages of radius update and reordering of the multiple SDAs to achieve great complexity reduction. Our SA-SSDA is the fastest one among the state-of-the-art algorithms to achieve the exact MLD of GenSM signals. Finally, for hardware implementation, the SA-SSDA scheme is inappropriate for hardware implementation, because it is of variable computational complexity. Instead, we consider the fixed-complexity tree search algorithms with nearly MLD performance to facilitate hardware implementation. Similar to the SA-SSDA, the sorting assisted successive K-best algorithm and reduced fixed complexity detector (rFSD) are studied. After performance evaluation, we determined to apply the rFSD successively and design a hardware architecture for detecting GenSM MIMO signals under the scenario of 5 transmit antennas, 2 transmit radio frequency chains, 4 receive antennas, and 64-QAM SMX symbols. Under the TSMC 90nm CMOS technology, the VLSI implementation of our GenSM detector (see Fig. 3) reveals that while operating at 322.6 MHz, our architecture requires 276.7K gates and provides detection throughput 1.613 Gbps. Compared with other related architectures, our architecture provides higher detection throughput rate and is of better hardware efficiency. In summary, we have developed the optimal MLD, in both algorithms and hardware architecture, for detecting the SM signals and GenSM signals. Our results provide state-of-the-art solutions to detect the SM and GenSM signals. The important results support the spatial modulation as a strong competitor for the future 5G and beyond.
STAY CONNECTED. SUBSCRIBE TO OUR NEWSLETTER.
Add your information below to receive daily updates.