Privacy Protection and Personal Information De-Identification for Artificial Intelligence Applications
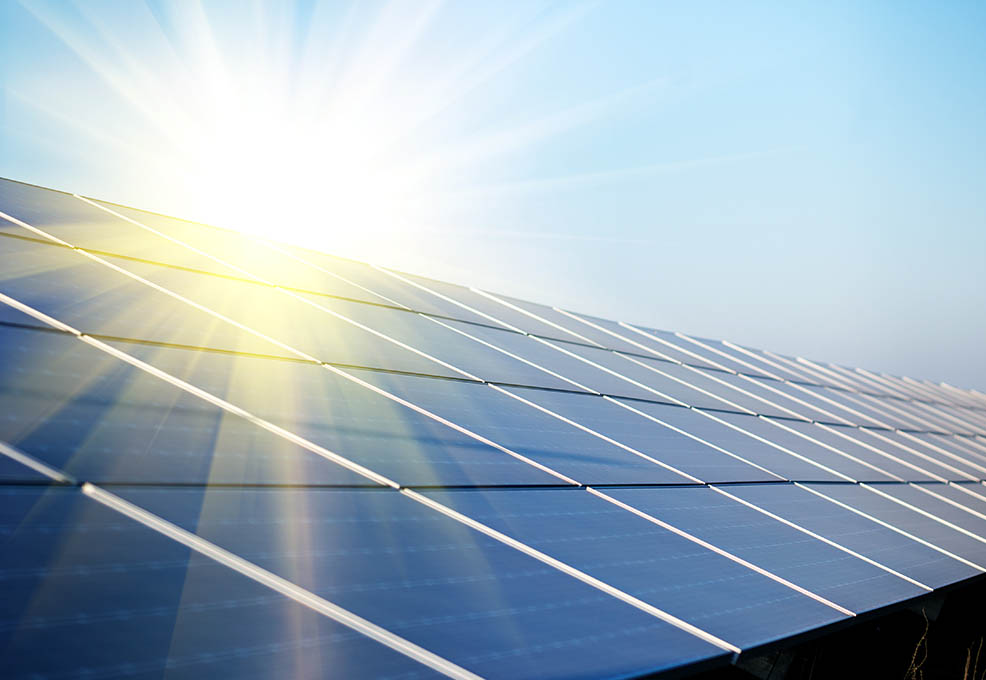
Author(s)
Kung-Hwa WeiBiography
Prof. Wei is currently in the Department of Material Science and Engineering at National Chiao Tung University (NCTU). His major research interests are in the areas of solar energy, 2D materials and highly efficient electronic devices. He has published more than 220 papers. He has accumulated about 15,873 citations for his papers, and his H-Index is ~64.
Academy/University/Organization
National Chiao Tung UniversitySource
https://www.sciencedirect.com/science/article/pii/S221128551730695X
https://pubs.acs.org/doi/10.1021/acs.nanolett.9b04586
https://pubs.acs.org/doi/abs/10.1021/acsnano.8b08577
https://onlinelibrary.wiley.com/doi/full/10.1002/adma.202003891
https://www.sciencedirect.com/science/article/pii/S2542435118305555-
TAGS
-
Share this article
You are free to share this article under the Attribution 4.0 International license
- ENGINEERING & TECHNOLOGIES
- Text & Image
- December 21,2020
Organic photovoltaics (OPVs) devices have attracted considerable attention in the last two decades. Non-fullerene acceptors (NFAs), novel polymer structures, and semitransparent and tandem cell structures have been adopted to prepare polymer solar cells displaying high power conversion efficiencies (PCEs). Here, Prof. Kung-Hwa Wei’s team in National Chiao Tung University, in collaboration with Prof. Yang Yang from UCLA, adopted four approaches to enhance the PCEs of OPVs. First, the application of ternary blends as the active layer for polymer solar cells can be tuned by varying the composition ratios of components in order to optimize the devices' PCEs. Using side-chain engineering to tune the structure of a minor conjugated polymer and, thus, influence another major conjugated polymer's packing provides highly efficient polymer cells. Second, the BHJ structure-based OPVs with the random mixing of donors and acceptors often suffers the severe charge recombination in the interface, resulting in poor charge extraction. Our team uses potassium ions to treat the zinc oxide electron transport layer's surface to induce the vertical phase separation of the polymer donor, and small molecule acceptors in the active layer such that a gradient concentration profile was produced for facilitating the carrier transport. Third, the visible and infrared (IR) regions comprise approximately 47% and 51% of the solar spectrum energy, respectively. Semi-transparent organic photovoltaics (TOPVs) based on IR-absorbing organic materials with two functions were adopted; they can utilize the IR light for electricity generation, and the penetrated visible light will be utilized for photosynthesis in plants. These results demonstrated TOPV devices with promising perspectives for building-integrated photovoltaics. Fourth, a tandem structure was developed to enhance photon utilization efficiency and reduce thermal loss with the unique advantages of NFAs. The combination with the tandem concept shows excellent potential for OPVs.
Figure 1. a building-incorporated semi-transparent organic photovoltaics with Infrared light for electric power generation and visible light for photosynthesis.
The application of ternary blends as the active layer for organic photovoltaics (OPVs)—for which the absorption spectra can be tuned by varying the composition ratios of components—is another facile approach toward optimizing the PCEs of devices. The selection of a suitable ternary blend active layer often relies on intuition and remains a formidable challenge. Here, we adopted a systemic approach of not only using the same donor chemical units in the two donor-acceptor (D/A) conjugated polymers with complementary light absorption, but also varying the side chains architecture as a means of tuning the packing of these semi-planar conjugated polymers, thereby influencing the carrier transport and optimizing the PCE. We employed linear, branch, and mixed linear-and-branch side-chain attached benzooxadiazole (BO) as the acceptor (A) units in poly [benzodithiophene-thiophene-benzooxadiazole] (PBDTTBO) conjugated polymers, and monitored their interactions with poly[benzodithiophene-fluorothienothiophene] (PTB7-TH), both of which featured the same benzodithiophene (BDTT) donor units. We found that incorporating a minor amount (10%) of D/A conjugated PBDTTBO with such side chains into the PTB7-TH with a fullerene allowed us to tune the packing of the two polymers and, thereby, enhance the PCEs of corresponding ternary blend devices; the PCE of the ternary blend device incorporating PBDTTBO with two branched-side chains, PTB7-TH, and PC71BM increased to 11.4% from 9.0% for the device incorporating only the binary blend of PTB7-TH and PC71BM—a relative increase of more than 25%. This approach of using side-chain engineering to tune the structure of a minor conjugated polymer and, thus, influence another major conjugated polymer's packing that features the same donor chemical units appears to provide highly efficient OPVs.
Figure 2. the power conversion efficiency (PCE) of the ternary blend device incorporating PBDTTBO, PTB7-TH and PC71BM increased to 11.4% from 9.0% for the device incorporating only the binary blend of PTB7-TH and PC71BM
Nano Energy 2018, 43, 138
Bulk heterojunction (BHJ) structure-based OPVs have recently shown great potential for achieving high PCEs. An ideal BHJ structure would feature a large D/A interfacial area for efficient exciton dissociation and gradient distributions with high donor and acceptor concentrations near the anode and cathode, respectively, for efficient charge extraction. However, the random mixing of donors and acceptors in the BHJ often suffers the severe charge recombination in the interface, resulting in poor charge extraction. Herein, we propose a new approach treating the zinc oxide (ZnO) surface as an electron transport layer with potassium hydroxide to induce vertical phase separation of an active layer incorporating the non-fullerene acceptor (NFA) IT-4F. Benefiting from efficient charge extraction, the best PCEs increased to 12.8% from 11.8% for PBDB-T-2Cl:IT-4F-based devices, to 12.6% from 11.6% for PBDB-T-2Cl:Y1-4F-based devices, to 13.5% from 12.2% for PBDB-T-2Cl:Y6-based devices, and to 15.7% from 15.1% for PM6:Y6-based devices.
Figure 3. As comparing devices incorporating potassium-treated electron transport ZnO layer with pristine ZnO layer, the PCEs increased to 13.5% from 12.2% for PBDB-T-2Cl:Y6-based devices, and to 15.7% from 15.1% for PM6:Y6-based devices.
Nano Lett. 2020, 20, 715
The visible and the infrared (IR) regions take up ∼47% and ∼51% of the energy in the solar spectrum (AM 1.5G standard), respectively, utilizing the visible light for plant growth and the IR light for power generation, which is potentially extremely exciting. IR absorbing organic semiconductors, with localized IR absorption and visible-light transmittance, would be promising materials for this purpose. Here, flexible semi-transparent organic photovoltaics (TOPVs) based on IR-absorbing organic materials were proposed, which can be a simple, low-cost, and promising way to utilize the IR light for electricity generation, while the penetrated visible light will be utilized for photosynthesis in plants. A power-conversion efficiency of ∼10% with an average visible transmittance of 34% was achieved for TOPV devices.
Figure 4. A semi-transparent organic photovoltaics with a PCE of ∼10% for electric power generation and an average visible light transmittance of 34% for photosynthesis was achieved for TOPV
ACS Nano 2019, 13, 1071
From the perspective of device engineering, almost all reported works reduce the thickness of the back/reflected electrode to obtain a high average visible transmittance (AVT), a trade-off between PCE and the transmittance, thus limiting further development. Herein, a unique strategy of “transparent hole-transporting frameworks” is proposed. A hole transporting large-bandgap polymer (poly[bis(4-phenyl)(2,4,6-trimethylphenyl) amine (PTAA)] is employed to partially replace polymer donors in the active layer of PBDB-T/Y1. PTAA is a p-type polymer with a large bandgap of 2.9 eV; the partial substitution of PBDB-T by PTAA reduces the active layer's absorption only in the visible region, keeping the hole-transporting pathways and the optimized film morphology. As a result, TOPVs with PCEs of 12% and AVTs of 20% are achieved, both on rigid and flexible substrates. These outcomes demonstrated TOPV devices with promising perspectives for building-integrated photovoltaics.
Figure 5. TOPVs with PCEs of 12% and AVTs of 20% are achieved when incorporating a hole transporting large-bandgap polymer (poly[bis(4-phenyl)(2,4,6-trimethylphenyl) amine
Adv. Mater. 2020, 32, 2003891
A tandem structure was invented to enhance photon utilization efficiency and reduce thermal loss. Considering the unique advantages of NFAs, the combination of NFAs and the tandem concept shows excellent potential for OPVs. Herein, a simple strategy to balance the voltage-current trade-off in tandem OPVs by employing mixed NFAs in rear sub-cells is reported. The VOC and JSC of rear sub-cells can be tuned using the blend of donor/NFA/NFA. This strategy offers a straightforward approach to balancing the VOC and JSC in tandem with OPVs toward higher PCE without rational and careful synthesis of new materials. Tandem OPVs based on mixed NFAs in rear sub-cells exhibited the best PCE of 13.3% in the lab. The National Renewable Energy Laboratory certified the tandem devices under asymptotic scans, and a PCE of 11.52% was achieved.
Figure 6. Tandem OPVs based on mixed NFAs in rear sub-cells exhibited the best PCE of 13.3%, and a NRL-certified PCE of 11.52% was achieved.
Joule 2019, 3, 1
STAY CONNECTED. SUBSCRIBE TO OUR NEWSLETTER.
Add your information below to receive daily updates.